In gas chromatography (GC) the gaseous mobile phase is forced through the staionary phase using pressure. A simple GC would include a tank of gas, pressure and flow regulators to control the gas flow, an oven, an injector to allow injection of a small volume of the sample mixture under pressure, a column containing the bed of stationary phase, a detector to detect the presence of components as they exit the column, and some means to record the detector signal.
A flow scheme for GC
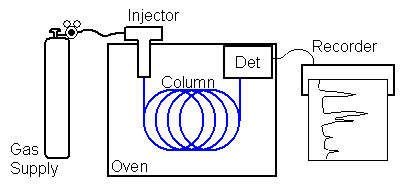
Retention timeThe time taken for a particular compound to travel through the column to the detector is known as its retention time. This time is measured from the time at which the sample is injected to the point at which the display shows a maximum peak height for that compound.Different compounds have different retention times. For a particular compound, the retention time will vary depending on:
-
the pressure used (because that affects the flow rate of the solvent)
the nature of the stationary phase (not only what material it is made of, but also particle size)
the exact composition of the solvent
-
the temperature of the column
That means that conditions have to be carefully controlled if you are using retention times as a way of identifying compounds.
Interpreting the output from the detector
Report the retention time of each peak (in minutes), the identity of each component in the mixture, and the percent composition of the mixture. To determine the percent composition, you will first need to find the area under each curve.
Area = (height) x (width at 1/2 height)
Mark retention time, height, half-height, and width at ? height on your GC trace.
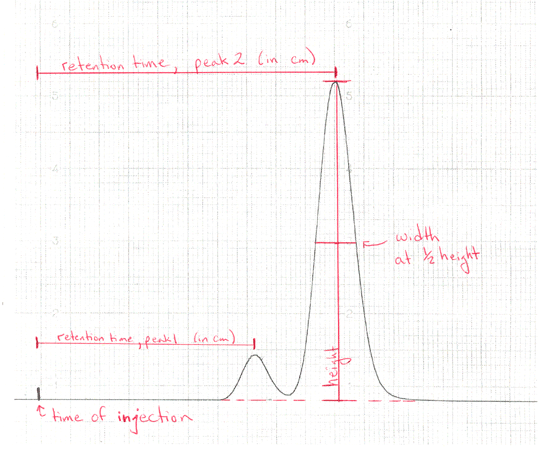
You may assume that each component of the mixture causes the same response in the detector. Therefore, the areas under the curves can be used to calculate percent composition of the mixture of alkenes. (This is a reasonable assumption when the components of the mixture are very similar in structure, as are 2-methyl-1-butene and 2-methyl-2-butene.)
% Component 1 = [(area under peak 1)/(total area)] x 100%
The sample used to obtain the GC trace that is shown above did not have any solvent in it. Actual samples will have at least one solvent present, so you will see another peak in your GC traces that typically appears very soon (usually within a minute) after injection. It is normal for the solvent peak to go off scale. |
Theory
A gas chromatograph consists of a flowing mobile phase, an injection
port, a separation column containing the stationary phase, a detector,
and a data recording system. The organic compounds are separated due to
differences in their partitioning behavior between the mobile gas phase
and the stationary phase in the column.
Mobile Phase
(Carrier gas)
The carrier gas must be chemically inert. Commonly used gases include nitrogen, helium, argon, and carbon dioxide. The choice of carrier gas is often dependant upon the type of detector which is used. The carrier gas system also contains a molecular sieve to remove water and other impurities.
Stationary Phase
The most common stationary phases in gas-chromatography columns are polysiloxanes, which contain various substituent groups to change the polarity of the phase. The nonpolar end of the spectrum is polydimethyl siloxane, which can be made more polar by increasing the percentage of phenyl groups on the polymer. For very polar analytes, polyethylene glycol (a.k.a. carbowax) is commonly used as the stationary phase. After the polymer coats the column wall or packing material, it is often cross-linked to increase the thermal stability of the stationary phase and prevent it from gradually bleeding out of the column.
Small gaseous species can be separated by gas-solid chromatography. Gas-solid chromatography uses packed columns containing high-surface-area inorganic or polymer packing. The gaseous species are separated by their size, and retention due to adsorption on the packing material.
INJECTIONPORT
The sample to be analyzed is loaded at the injection port via a hypodermic syringe.The injection port is heated in order to volatilize the sample.Once in the gas phase, the sample is carried onto the column by the carrier gas, typically helium.The carrier gas is also called the mobile phase.Gas chromatographs are very sensitive instruments.Typically samples of one microliter or less are injected on the column.These volumes can be further reduced by using what is called a split injection system in which a controlled fraction of the injected sample is carried away by a gas stream before entering the column.
Detector
If the column conditions are chosen correctly, the components in the sample will exit the column and flow past the detector one at a time.There are several different types of detectors common to gas chromatography instruments.The choice of detector is determined by the general class of compounds being analyzed and the sensitivity required.Flame ionization detectors (FIDs) are the most widely used detectors for organic samples.FIDs use an air/hydrogen flame to pyrolyze the effluent sample.The pyrolysis of the compounds in the flame creates ions.A voltage is applied across the flame and the resulting flow of ions is detected as a current.The number of ions produced, and therefore the resulting current, depends on the flame conditions and the identity of the molecule in question.(As a rough approximation, the current is proportional to the number of reduced carbons in the molecule.)In other words, the detector shows a different response to each compound.For this reason, separate calibrations must be performed for each compound analyzed.
1. Mass Spectrometer (GC/MS)
Many GC instruments are coupled with a mass spectrometer, which is a very good combination. The GC separates the compounds from each other, while the mass spectrometer helps to identify them based on their fragmentation pattern.
2. Flame Ionization Detector (FID)
The detector is very sensitive towards organic molecules (10-12 g/s, linear range: 106 ¨C107), but relative insensitive to a few small molecules e.g. N2, NOx, H2S, CO, CO2, H2O. If proper amounts of hydrogen/air are mixed, the combustion does not afford any ions. If other components are introduced that contain carbon atoms cations are produced in the effluent stream. The more carbon atoms are in the molecule, the more fragments are formed and the more sensitive the detector is for this compound (-- > response factor). However, due to the fact that the sample is burnt (pyrolysis), this technique is not suitable for preparative GC. In addition, several gases are usually required to operate a FID: hydrogen, oxygen (compressed air), and carrier gas.
3. Thermal Conductivity Detector (TCD)
This detector is less sensitive than the FID (10-5-10-6g/s, linear range: 103-104), but is well suited for preparative applications, because the sample is not destroyed. It is based on the comparison of two gas streams, one containing only the carrier gas, the other one the carrier gas and the compound. Naturally, a carrier gas with a high thermal conductivity e.g. helium or hydrogen is used in order to maximize the temperature difference (and therefore the difference in resistance) between two thin tungsten wires. The large surface-to-mass ratio permits a fast equilibration to a steady state. The temperature difference between the reference cell and the sample cell filaments is monitored by a Wheatstone bridge circuit.
4. Electron Capture Detector (ECD)
The detector consists of a cavity that contains two electrodes and a radiation source that emits b-radiation (e.g. 63Ni, 3H). The collision between electrons and the carrier gas (methane plus an inert gas) produces a plasma containing electrons and positive ions. If a compound is present that contains electronegative atoms, those electrons are ¡°captured¡± and negative ions are formed, and the rate of electron collection decreases. The detector is extremely selective for compounds with atoms of high electron affinity (10-14 g/s), but has a relatively small linear range (~102-103). This detector is frequently used in the analysis of chlorinated compounds e.g. pesticides, polychlorinated biphenyls, which show are very high sensitivity.
Columns
There are two general types of column, packed and capillary (also known as open tubular). Packed columns contain a finely divided, inert, solid support material (commonly based on diatomaceous earth) coated with liquid stationary phase. Most packed columns are 1.5 - 10m in length and have an internal diameter of 2 - 4mm.
Capillary columns have an internal diameter of a few tenths of a millimeter. They can be one of two types; wall-coated open tubular (WCOT) or support-coated open tubular (SCOT). Wall-coated columns consist of a capillary tube whose walls are coated with liquid stationary phase. In support-coated columns, the inner wall of the capillary is lined with a thin layer of support material such as diatomaceous earth, onto which the stationary phase has been adsorbed. SCOT columns are generally less efficient than WCOT columns. Both types of capillary column are more efficient than packed columns.
In 1979, a new type of WCOT column was devised - the Fused Silica Open Tubular (FSOT) column;
These have much thinner walls than the glass capillary columns, and are given strength by the polyimide coating. These columns are flexible and can be wound into coils. They have the advantages of physical strength, flexibility and low reactivity.
The column is where the components of the sample are separated.The column contains the stationary phase.Gas chromatography columns are of two types¡ªpacked and capillary.Capillary columns are those in which the stationary phase is coated on the interior walls of a tubular column with a small inner diameter.
For example, We will use a capillary column in this experiment.
The stationary phase in our column is a polysiloxane material.The basic structure of the polymeric molecules is shown below, where n indicates a variable number of repeating units and R indicates an organic functional group.In ourcolumns, 5% of the ¡°R¡¯s¡± are methyl groups (-CH3) and 95% of the ¡°R¡¯s¡± are phenyl groups (-C6H5)
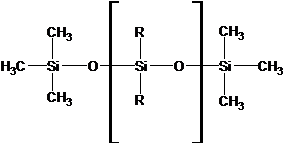
This polymeric liquid has a high boiling point that prevents it from evaporating off the column during the experiment.
The components in the sample get separated on the column because they take different amounts of time to travel through the column depending on how strongly they interact with the stationary phase.As the components move into the column from the injection port they dissolve in the stationary phase and are retained.Upon re-vaporization into the mobile phase they are carried further down the column.This process is repeated many times as the components migrate through the column.Components that interact more strongly with the stationary phase spend proportionally less time in the mobile phase and therefore move through the column more slowly.Normally the column is chosen such that it¡¯s polarity matches that of the sample.When this is the case, the interaction and elution times can be rationalized according to Raoult¡¯s law and the relationship between vapor pressure and enthalpy of vaporization.The rule of thumb is that retention times correlate with boiling points.(Do not expect an exact quantitative correlation, i.e. one with an R-value close to one, for this simple model.You will be using a non-polar column and the interaction between an alcohol molecule and the stationary phase will be dominated by weak van der Waals forces.)
What influences the separation?
1. Polarity of the stationary phase
Polar compounds interact strongly with a polar stationary phase, hence have a longer retention time than non-polar columns. Chiral stationary phases based on amino acid derivatives, cyclodextrins, chiral silanes, etc are capable to separate enantiomers, because one form is slightly stronger bonded than the other one, often due to steric effects.
2. Temperature
The higher the temperature, the more of the compound is in the gas phase. It does interact less with the stationary phase, hence the retention time is shorter, but the quality of separation deteriorates.
3. Carrier gas flow
If the carrier gas flow is high, the molecules do not have a chance to interact with the stationary phase. The result is the same as above.
4. Column length
The longer the column is the better the separation usually is. The trade-off is that the retention time increases proportionally to the column length. There is also a significant broadening of peaks observed, because of increased back diffusion inside the column.
5. Amount of material injected
If too much of the sample is injected, the peaks show a significant tailing, which causes a poorer separation. Most detectors are relatively sensitive and do not need a lot of material (see below).
6. Conclusion
High temperatures and high flow rates decrease the retention time, but also deteriorate the quality of the separation.
-------------
Manufacturers
|