Instrumentation:
In order to measure the characteristics of individual molecules, a mass spectrometer converts them to ions so that they can be moved about and manipulated by external electric and magnetic fields.
In general a mass spectrometer consists of:
1) an ion source
2) a mass-selective analyzer
3)an ion detector.
Since mass spectrometers create and manipulate gas-phase ions, they operate in a high-vacuum system. The magnetic-sector, quadrupole, and time-of-flight designs also require extraction and acceleration ion optics to transfer ions from the source region into the mass analyzer. The details of mass analyzer designs are discussed in the individual documents listed below. Basic descriptions of sample introduction/ionization and ion detection are discussed in separate documents on ionization methods and ion detectors, respectively.
A flow scheme for MS
When a high energy electron collides with a molecule it often ionizes it by knocking away one of the molecular electrons (either bonding or non-bonding). This leaves behind a molecular ion (colored red in the following diagram). Residual energy from the collision may cause the molecular ion to fragment into neutral pieces (colored green) and smaller fragment ions (colored pink and orange). The molecular ion is a radical cation, but the fragment ions may either be radical cations (pink) or carbocations (orange), depending on the nature of the neutral fragment. An animated display of this ionization process will appear if you click on the ion source of the mass spectrometer diagram.

Theory
Sample Introduction
The selection of a sample inlet depends upon the sample and the sample matrix. Most
ionization techniques are designed for gas phase molecules so the inlet must transfer the analyte
into the source as a gas phase molecule. If the analyte is sufficiently volatile and thermally
stable, a variety of inlets are available. Gases and samples with high vapor pressure are
introduced directly into the source region. Liquids and solids are usually heated to increase the
vapor pressure for analysis. If the analyte is thermally labile (it decomposes at high
temperatures) or if it does not have a sufficient vapor pressure, the sample must be directly
ionized from the condensed phase. These direct ionization techniques require special
instrumentation and are more difficult to use. However, they greatly extend the range of
compounds that may be analyzed by mass spectrometry. Commercial instruments are available
that use direct ionization techniques to routinely analyze proteins and polymers with molecular
weights greater than 100,000 dalton.
Direct Vapor Inlet. The simplest sample introduction method is a direct vapor inlet. The
gas phase analyte is introduced directly into the source region of the mass spectrometer through a
needle valve. Pump out lines are usually included to remove air from the sample. This inlet
works well for gases, liquids, or solids with a high vapor pressure. Samples with low vapor
pressure are heated to increase the vapor pressure. Since this inlet is limited to stable compounds
and modest temperatures, it only works for some samples.
Gas Chromatography. Gas chromatography is probably the most common technique for
introducing samples into a mass spectrometer. Complex mixtures are routinely separated by gas
chromatography and mass spectrometry is used to identify and quantitate the individual
components. Several different interface designs are used to connect these two instruments. The
most significant characteristics of the inlets are the amount of GC carrier gas that enters the mass
spectrometer and the amount of analyte that enters the mass spectrometer. If a large flow of GC
carrier gas enters the mass spectrometer it will increase the pressure in the source region.
Maintaining the required source pressure will require larger and more expensive vacuum pumps.
The amount of analyte that enters the mass spectrometer is important for improving the detection
limits of the instrument. Ideally all the analyte and none of the GC carrier gas would enter the
source region.
The most common GC/MS interface now uses a capillary GC column. Since the carrier
gas flow rate is very small for these columns, the end of the capillary is inserted directly into the
source region of the mass spectrometer. The entire flow from the GC enters the mass
spectrometer. Since capillary columns are now very common, this inlet is widely used.
However, wide bore capillaries and packed GC columns have higher flow rates. This
M is the molecular ion produced by removing a single electron to f * + orm a radical cation. M is the
molecule, + is the charge of the cation, and is the remaining unpaired electron of the radical.
**Adduct ions are produced by a chemical reaction between an ion and a neutral molecule. Many of these
reactions cause the addition of a proton (H+) to the molecule (M) and produce an adduct ion (MH ). +
6
significantly increases the pressure in the mass spectrometer. Several inlet designs are available
to reduce the gas flow into the source. The simplest design splits the GC effluent so that only a
small portion of the total flow enters the mass spectrometer. Although this inlet reduces the gas
load on the vacuum system, it also reduces the amount of analyte. Effusive separators and
membrane inlets are more selective and transport a higher fraction of the analyte into the source
region. Each of these methods has efficiency and resolution drawbacks but they are necessary for
some experiments.
Liquid Chromatography. Liquid chromatography inlets are used to introduce thermally
labile compounds not easily separated by gas chromatography. These inlets have undergone
considerable development and are now fairly routine. Because these inlets are used for
temperature sensitive compounds, the sample is ionized directly from the condensed phase.
These inlets are discussed in greater detail in the section on ionization techniques.
Direct Insertion Probe. The Direct Insertion Probe (DIP) is widely used to introduce low
vapor pressure liquids and solids into the mass spectrometer. The sample is loaded into a short
capillary tube at the end of a heated sleeve. This sleeve is then inserted through a vacuum lock
so the sample is inside the source region. After the probe is positioned, the temperature of the
capillary tube is increased to vaporize the sample. This probe is used at higher temperatures than
are possible with a direct vapor inlet. In addition, the sample is under vacuum and located close
to the source so that lower temperatures are required for analysis. This is important for analyzing
temperature sensitive compounds. Although the direct insertion probe is more cumbersome than
the direct vapor inlet, it is useful for a wider range of samples.
Direct Ionization of Sample. Unfortunately, some compounds either decompose when
heated or have no significant vapor pressure. These samples may be introduced to the mass
spectrometer by direct ionization from the condensed phase. These direct ionization techniques
are used for liquid chromatography/mass spectrometry, glow discharge mass spectrometry, fast
atom bombardment and laser ablation. The development of new ionization techniques is an
active research area and these techniques are rapidly evolving. Direct ionization is discussed in
greater detail in the next section.
IONIZATION TECHNIQUES:
CI uses a reagent ion to react with the analyte molecules to form ions by either a proton or hydride transfer:
MH + C2H5+ --> MH2+ + C2H4
MH + C2H5+ --> M+ + C2H6
The reagent ions are produced by introducing a large excess of methane (relative to the analyte) into an electron impact (EI) ion source. Electron collisions produce CH4+ and CH3+ which further react with methane to form CH5+ and C2H5+:
CH4+ + CH4 --> CH5+ + CH3
CH3+ + CH4 --> C2H5+ + H2
A plasma is a hot, partially-ionized gas that effectively excites and ionizes atoms. The most common plasma source is an inductively-coupled plasma.
A glow discharge is a low-pressure plasma maintained between two electrodes. It is particularly effective at sputtering and ionizing material from solid surfaces.
An EI source uses an electron beam, usually generated fron a tungsten filament, to ionize gas-phase atoms or molecules. An electron from the beam knocks an electron off of analyte atoms or molecules to create ions.
The ESI source consists of a very fine needle and a series of skimmers. A sample solution is sprayed into the source chamber to form droplets. The droplets carry charge when the exit the capillary and, as the solvent evaporates, the droplets disappear leaving highly charged analyte molecules. ESI is particularly useful for large biological molecules that are difficult to vaporize or ionize.
In FAB a high-energy beam of netural atoms, typically Xe or Ar, strikes a solid or low-vapor-pressure liquid sample causing desorption and ionization. It is used for large biological molecules that are difficult to get into the gas phase. The sample is usually dispersed in a matrix such as glycerol. FAB causes little fragmentation and usually gives a large molecular ion peak, making it useful for molecular weight determination.
The atomic beam is produced by accelerating ions from an ion source though a charge-exchange cell. The ions pick up an electron in collisions with netural atoms to form a beam of high-energy atoms.
Molecules can lose an electron when placed in a very high electric field. High fields can be created in an ion source by applying a high voltage between a cathode and an anode called a field emitter. A field emitter consists of a wire covered with microscopic carbon dendrites, which greatly amplify the effective field at the carbon points.
A laser pulse ablates material from the surface of a sample, and creates a microplasma that ionizes some of the sample constituents. The laser pulse accomplishes both vaporization and ionization of the sample.
MALDI is a LIMS method of vaporizing and ionizing large biological molecules such as proteins or DNA fragments. The biological molecules are dispersed in a solid matrix such as nicotinic acid or dihydroxybenzoic acid.
A UV laser pulse ablates the matrix which carries some of the large molecules into the gas phase in an ionized form so they can be extracted into a mass spectrometer.
Decay of 252Cf produces two fission fragments that travel in opposite directions. One fragment strikes the sample knocking out 1-10 analyte ions. The other fragment strikes a detector and triggers the start of data acquisition. This ionization method is especially useful for large biological molecules.
Resonance ionization (RIMS)
One or more laser beams are tuned in resonance to transistions of a gas-phase atom or molecule to promote it in a stepwise fashion above its ionization potential to create an ion. Solid samples must be vaporized by heating, sputtering, or laser ablation.
A primary ion beam; such as 3He+,16O+, or 40Ar+; is accelerated and focused onto the surface of a sample and sputters material into the gas phase. Approximately 1% of the sputtered material comes off as ions, which can then be analyzed by a mass spectrometer. SIMS has the advantage that material can be continually sputtered from a surface to determine analyte concentrations as a function of distance from the original surface (depth profiling).
A spark source ionizes analytes in solid samples by pulsing an electric current across two electrodes. If the sample is a metal it can serve as one of the electrodes, otherwise it can be mixed with graphite and placed in a cup-shaped electrode.
Thermal ionization is used for elemental or refractory materials. A sample is deposited on a metal ribbon, such as Pt or Re, and an electric current heats the metal to a high temperature. The ribbon is often coated with graphite to provide a reducing effect.
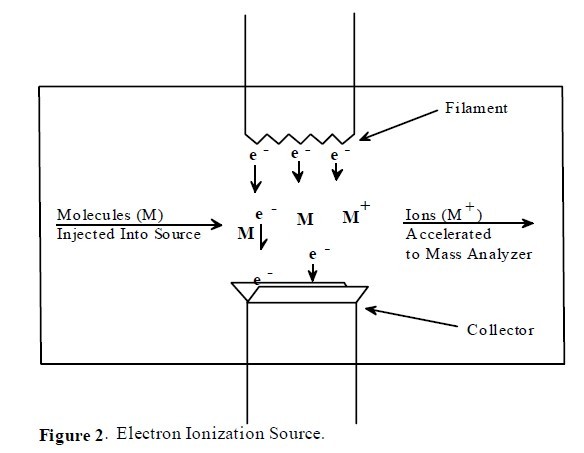
|